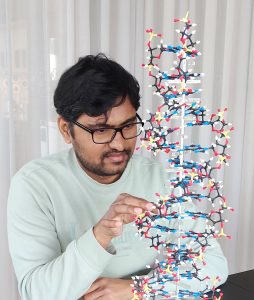
Tharun Kumar Kotammagari
TCSMT Postdoctoral Research Fellow
Bioorganic group
Department of Chemistry
University of Turku
For our body to function, we need to supply it with a variety of nutrients that we get from our diet. However, our bodies can not use the food as it is when it enters our digestive system. The process of chemical digestion uses different proteins and enzymes to break down large molecules into usable nutrients that our cells absorb. Where are the instructions to manufacture these and all types of proteins we need to stay alive? The instructions to make proteins are contained within our DNA.
DNA is a biopolymer that stores the genetic information in the sequence of four nucleic acid bases – adenine (A), thymine (T), cytosine (C), and guanine (G). These bases are strung along with the ribbons of a deoxyribose sugar-phosphate backbone and form a double-helical structure with the help of hydrogen bonding. The above-mentioned proteins and Watson-Crick base pairing properties are well established in the literature. Metal ions such as K+, Na+, and Mg2+ are also responsible for catalyzing the reactions of metabolism and the correct folding of biopolymers (nucleic acids). Generally, the sugar-phosphate backbone carries a negative charge and these charges are partially neutralized by the aforementioned alkali and alkaline earth metals. These metal ions also play a key role in the central dogma of biology (replication, transcription, and translation). However, less attention is given to the inorganic components of a biological cell, which are required for a biopolymer to function.
Metal mediated base pairs form by replacing the hydrogen bonds between complementary nucleobases in DNA with coordinate bonds formed by the metals, especially transition metals. Coordinate bonds have more strength than the H-bonds and transition metals readily form coordinate bonds with nucleobases. Due to this DNA duplexes possessing metal-mediated base pairs show higher thermal stability than the natural H-bonded DNAs.
Covalent bonds are even stronger than the coordinate and hydrogen bonds. What if one or more H-bonds or coordinate bonds are replaced with covalent bonds in the respective duplexes? The duplex stability will definitely increase. With this idea, covalently mercurated oligonucleotides were synthesized and examined for their duplex stability. In almost all the cases, greater duplex stability was observed. This idea was extended further to recognize sulfur-modified nucleosides. Sulfur modifications have been frequently discovered on transfer RNAs (tRNAs). These modifications play an important role and are found in all types of organisms. Given the high affinity of mercury and sulfur, one can expect greater duplex stability. When covalently mercurated nucleobases are placed opposite to the sulfur-containing nucleobases extremely higher thermal duplex stability have been observed.
Oligonucleotide therapeutics has emerged as a promising field in recent years. The antisense oligonucleotides (also known as ASOs or AONs) are small molecules that can be used to either inhibit or alter the protein synthesis. However, the delivery of the ASOs to disease sites and across cell membranes is still a major challenge for gene regulation therapies (antisense DNA and siRNA). So far various ASOs delivery strategies are reported. Among them, spherical nucleic acids (SNA)−Nano Particle (NP) conjugates is one of the effective strategies focused on by the bioorganic chemistry group at the University of Turku. These SNA-NP conjugates have many advantages as they are specific for intracellular applications and have high binding coefficients for complementary DNA and RNA. In addition, the advantages are nuclease resistance, no observed toxicity, and highly effective gene- regulating capabilities. We have recently started a new research project to adsorb covalently metallated oligonucleotides on the surface of the different types of nanoparticles. These adsorbed oligonucleotides will be evaluated for the intracellular delivery and sensing of different sequences in the new cell lab recently set up in the chemistry department.